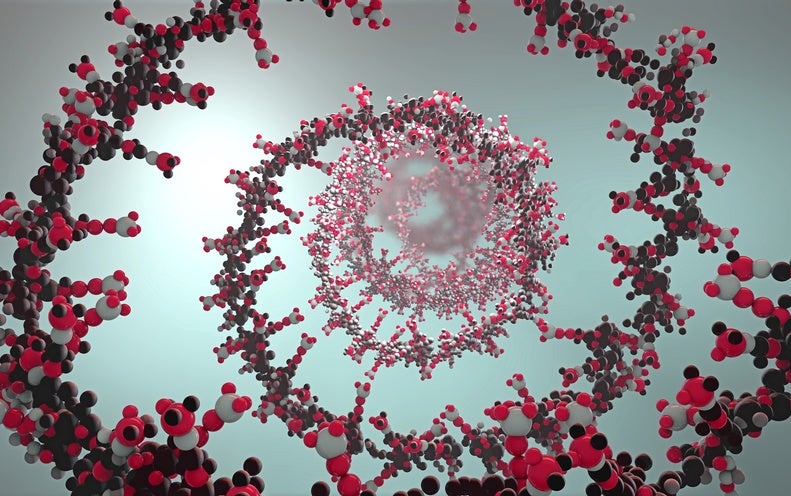
DNA and RNA, the two major modern forms of genetic code underpinning all of earthly biology, could have coexisted in strict pairings on our planet before life arose here, scientists in England, Scotland and Poland say. Using a hydrogen cyanide–based chemical system intended to mimic conditions in Earth’s early history, the researchers made four bases, the molecular “letters” of the genetic alphabet. Strung together, these bases form gene sequences that cells translate into proteins. But surprisingly, the team found that of the four bases their experiments consistently made, two were in a form found in DNA, whereas the other two were of a kind seen in RNA.
The study, published in Nature and conducted by John Sutherland of the Medical Research Council Laboratory of Molecular Biology in Cambridge, England, and his colleagues, further undermines the so-called RNA world hypothesis. This idea, long one of the most prominent in origins-of-life research, posits that RNA formed the basis of Earth’s biosphere long before DNA and other molecules important to life emerged. Yet to date, scant evidence has been found of chemical pathways to make the RNA-exclusive system that rigid versions of the idea adopt or that could lead to DNA. “People have tended to think of RNA as the parent of DNA,” Sutherland says. “This [paper] suggests that they are molecular siblings.”
Other scientists who were not involved with the study question the plausibility of the conditions used in this hydrogen cyanide-based route, however. Frances Westall, director of the exobiology group at the French National Center for Scientific Research’s Center for Molecular Biophysics in Orléans, notes that forming the bases requires very specific conditions. Mixtures would need to dry out and be exposed to ultraviolet light—two hurdles most easily surmounted on dry land, which was in short supply during our planet’s ocean-covered early days more than four billion years ago. “These conditions certainly existed on the early Earth,” Westall says. “They would not have been that common because there was not that much exposed landmass.” Although she adds that the study is “clever” and “not completely impossible,” she concludes that “there are other, better hypotheses as to locations for the emergence of life and prebiotic molecules.”
Arguments about plausibility have plagued the chemistry-based quest to understand life’s beginnings on Earth since the early 1950s, when American researchers Stanley Miller and Harold Urey performed a landmark experiment. The pair simulated the effects of lightning in the early Earth’s atmosphere and ocean by triggering electrical discharges in flasks containing hydrogen, water, ammonia and methane. Although their experiment famously produced sizable organic molecules vital for biochemistry, for decades other researchers have debated the plausibility of its conditions. Nevertheless, Miller and Urey’s work showed that it was relatively simple to make important substances, such as the amino acids that link up to form proteins that perform myriad functions within living cells. Of particular relevance to origins-of-life studies, proteins can act as catalysts, enhancing and speeding up other chemical reactions that would otherwise be too slow or inefficient to plausibly occur. But proteins are not the only possible catalysts behind the rise of life on Earth.
In work that would ultimately net the 1989 Nobel Prize in Chemistry, molecular biologist Sidney Altman and biochemist Thomas Cech found that RNA—long considered merely an intermediate carrier of genetic information that is subservient to DNA—can also behave as a catalyst. The RNA world hypothesis suggests that such molecules could self-replicate, enabling early evolution before the existence of DNA and proteins. The idea, however, “was an overzealous, overenthusiastic response to a brilliant discovery,” Sutherland says.
That response might have come partly because, to a naive chemist, it looks easy to leap from RNA to DNA. To create the long chains we often see coiled up into the DNA’s iconic double helix, the bases are first connected to a “backbone” of sugar molecules. These combinations make up nucleosides: deoxyribonucleotides in DNA and ribonucleosides in RNA—which, unlike its DNA cousin, forms a single helix. The nucleosides do not use table sugar, or sucrose, but rather ribose in RNA and deoxyribose in DNA (the different sugars give each material its first initial). The distinction between the two sugar types is tiny: just one oxygen and one hydrogen atom. Yet that difference is enough for DNA and RNA to have distinct biological roles. And biochemically removing the atoms is far harder than simply erasing the letters representing them in a notebook.
Another flaw in the RNA world idea has been the difficulty of making ribose in the conditions that probably existed on the early Earth—and to then connect it to a base. Sutherland and his colleagues therefore sought more likely ways to make ribose sugars and ribonucleosides. One of their most promising approaches relied on two gases thought to have been relatively abundant in the planet’s early atmosphere: hydrogen sulfide and hydrogen cyanide. When dissolved in water, bathed in ultraviolet light and subjected to cycles of drying, these simple compounds have produced many more complex molecules. They include amino acids and glycerol, the backbones of fatty molecules that can form cells’ outer wall.
Sutherland took this approach a step further last year. Working with Ramanarayanan Krishnamurthy’s team at the Scripps Research Institute in La Jolla, Calif., he and his colleagues showed that the ribonucleosides cytidine and uridine could be transformed into deoxyribose and the nucleoside and deoxyadenosine. Now—primarily via the efforts of team members Jianfeng Xu and Václav Chmela, both then at the Laboratory of Molecular Biology—the researchers have made even more progress. They mixed some of the intermediate molecules from the team’s previous studies with salts such as sodium nitrite and magnesium chloride that could have been prevalent on the primordial Earth, then subjected them to acidic conditions and heat, respectively. Through these steps, the scientists found two possible routes to add a fourth base, the less common nucleoside inosine, to their preexisting collection. The addition was enough to make a four-letter genetic alphabet in which each base in a strand would exclusively pair with one of the other three letters in a second strand That base-pairing complementarity is how modern RNA and DNA works. But in the experiment, two letters came from RNA, and two came from DNA.
The arrangement “suggests that the chemistry to make RNA and DNA isn’t as different as people have thought,” Sutherland says. “People have tended to think of RNA coming before DNA and somehow then being taken over. This, to me, is suggesting that it’s possible that you could have had an RNA-DNA hybrid, which could then give rise to the two separate molecules.” Sutherland’s team has not yet assembled the individual nucleosides and ribonucleosides into longer chains, however. Doing so is important, because showing that hybrid strands can really form and bind to a partner strand is crucial for moving the idea beyond speculation.
This is a key issue for Nicholas Hud, an origins-of-life researcher at the Georgia Institute of Technology, who was not involved in the study. He calls it an “excellent compilation of organic chemistry research” on water-based nucleoside synthesis. But Hud is not convinced that the paper resolves whether these nucleosides actually arose before living creatures. His own research suggests amino acids could have linked up to carry information and act as a catalyst before RNA. Hud thinks evolution would then have gradually produced the current genetic system over long stretches of geologic time. “If a molecule looks very difficult, from a chemical perspective, to make, yet it functions exquisitely in biology, then it’s probably the case that it has been evolved over time,” he says. For the same reasons, he is also skeptical about the RNA world hypothesis.
Furthermore, Hud sees the new study’s reliance on rigid incremental steps, each performed in strict order and under carefully controlled conditions, as a significant weakness. If the order of the steps changed or certain products were not isolated, Sutherland and his colleagues would have made much less of the substances they are interested in, Hud says. That caveat reduces the chances of the scenario unfolding in the chaotic environs of the early Earth.
Sutherland admits that, absent a time machine to travel back to life’s true origins on our planet, “plausibility is everything” in this rarefied field of research. Even so, he firmly backs his team’s work on establishing chemical routes to life’s building blocks. “There are many, many fingers pointing at hydrogen cyanide,” Sutherland asserts. “Does it prove that it all happened from hydrogen cyanide? It doesn’t prove it. But it’s good enough for me.”
"first" - Google News
June 22, 2020 at 06:15PM
https://ift.tt/2YkJaRY
The First Organism on Earth May Have Been a Genetic Hybrid - Scientific American
"first" - Google News
https://ift.tt/2QqCv4E
https://ift.tt/3bWWEYd
Bagikan Berita Ini
0 Response to "The First Organism on Earth May Have Been a Genetic Hybrid - Scientific American"
Post a Comment