A new study by scientists from the University of California San Diego, USA and Maynooth University, Ireland and published on the preprint server bioRxiv* in June 2020 reports preliminary results showing that the spike protein of the SARS-CoV-2, as well as its glycan coat, has “hitherto unseen functional and structural” properties. These may be useful in designing novel therapeutics to target this molecule.
Even as the COVID-19 pandemic continues to circulate, causing hundreds of thousands of deaths the world over, scientists strive to develop an effective vaccine or therapeutic. However, most efforts are directed at the spike protein of the virus.
.jpg)
The S Protein
Like other coronaviruses, the SARS-CoV-2 has a lipid envelope and a set of structural proteins forming a scaffold and envelope, along with the host cell membrane, for the nucleic acid of the virus. The S or spike protein is trimeric, with three domains, the head, stalk, and cytoplasmic tail.
Each protomer has an S1 and S2 subunit that makes up the head region. There are two cleavage sites, one for the transmembrane serine protease TMPRSS2 at the interface of S1 and S2, which is essential to prime and activate the spike protein so that infection can occur, and another for furin. The second cleavage site at S2 releases the fusion peptide, which prepares the host cell membrane for fusion.
The head of the spike has multiple domains, one being an N-terminal domain and another the receptor-binding domain (the NTD and the RBD respectively). The RBD has a receptor-binding motif (RBM) that interacts with the receptor ACE2 molecule on the host cell membrane to enter the cell. The S2 is “a metastable spring-loaded fusion machine” that brings about host cell and viral membrane fusion, and consists of the fusion peptide, and other domains.
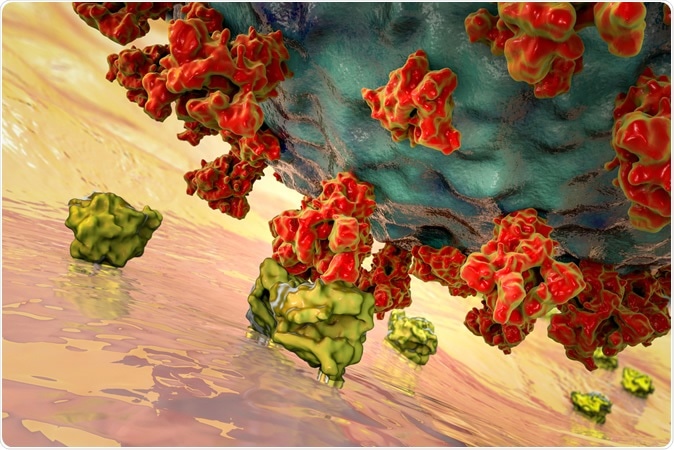
Glycosylation and Viral Function
Beyond all these domains is the heavy glycosylation of the S protein that is nonetheless difficult to characterize in detail. This modification is one that is essential to the disease-causing process, as demonstrated in the thick coating of N-glycans on the viral fusion proteins of many viruses. For instance, the HIV-1 has an envelope spike, which is almost completely covered with N-glycan over the whole of the exposed area. The N-glycans make up over half the molecular weight of the protein.
These N-glycans have a range of functions, because of their chemical structure. For instance, the glycans on the viral envelope often recognized host cell receptors and initiate viral entry via membrane fusion.
Another possible role is shielding the viral proteins from the host immune system, by coating the exposed protein surfaces with glycoproteins that are non-immunogenic, or only weakly so. The envelope glycan on the HIV-1 virus is mostly composed of oligomannose, which is perfect for this purpose.
On the other hand, the coronaviruses SARS and MERS have less well-shielded S proteins. They have a different glycosylation pattern, with more complex N-glycans compared to the oligomannose of the HIV-1 virus.
Each of the protomers that make up the SARS-CoV-2 trimer is predicted to have 22 N-glycosylation sites, 17 being occupied, with 2 O-glycosylation sites.
Glycans Shield Spike Protein
The current paper focuses on molecular dynamics (MD) simulations of the whole atom, showing the glycosylation profile of the whole S glycoprotein embedded in the virus membrane. This provides an insight into how the RBM becomes more or less accessible as the RBD conformation is ‘up’ or ‘down’. This emphasizes the role played by the glycans in the recognition and binding of ACE2 and thus for host cell invasion.
The simulation of the full length of the S protein shows that the stalk is almost completely shielded, especially for large molecules, including antibodies. However, the head region presents many more accessible epitopes and would be a better target for antibody development.
Glycosylation Coordinates with Conformational Changes
Finally, the researchers show that the special glycosylation pattern of the S protein on the SARS-CoV-2 is also an intrinsic functional part of the molecule. Certain sugars at specific locations play a modulatory role on the conformation of the S protein so that the RBD remains stable in the ‘up’ position.
The N-glycans on the RBM of the S protein head must both protect it from immune recognition but allow it to recognize and bind to the ACE2 receptors since this is the primary route of host cell entry. This demands that the glycans coordinate with the conformational changes.
The researchers describe this relationship: “This suggests that the glycan shield of this critical domain is effectively paired with its “down-to-up” conformational change, allowing the RBM to transiently emerge from the glycan shield and bind to ACE2 receptors.”
Beyond shielding, simulations predict glycans "lock and load" the #SARSCoV2 spike protein for infection @LCasalino88 @zied_gaieb @ElisaTelisa @abbydommer @biobryn #gotglycans? #glycotime #COVID19https://t.co/7ePW0Z5Uvx pic.twitter.com/4JNF6RbjGI
— Rommie Amaro (@RommieAmaro) June 12, 2020
Glycosylation Shields Epitopes Against Antibodies
The area of the RBM is, therefore, much more extensively covered by glycans in the ‘up’ conformation than in the ‘down’ conformation, at 35% vs. 9%, respectively. When a larger molecule, such as an antibody, is used to probe the accessibility of the RBM, this difference was still more marked, at 46% vs. 11%. With smaller probes, the shielding effect is much weaker, at 6% and 16%, respectively, for ‘up’ and ‘down’ configurations.
This variability is not seen in the rest of the RBD region away from the RBM, which is shielded in both conformations by glycans. These N-glycans are bound to the RBD at critical residues. The final picture, therefore, reveals an accessible RBM in the ‘up’ conformation of the RBD, but one which is heavily camouflaged in the ‘down’ position.
On the other hand, antibodies may not be able to penetrate the RBD in the ‘down’ position, but small-molecule drugs can do so, the simulation suggests. Structural studies performed earlier confirm that this is the case, showing that host antibodies can only neutralize the C-terminal domain-1 if the RBD is ‘up.’
The researchers also describe a number of antibodies directed against the S protein, most of which target the RBD epitopes, but a few against regions in the NTD and CD. These are minimally shielded by glycans in the ‘up’ configuration, and this is taken advantage of by antibodies to bind the S protein at this point. The shielding effect is much higher, from 11%/13% to 47%/62% or 38%/49% depending on the epitope and the size of the probe. Finally, with the RBD ‘down’, the RBM is buried within the cave formed by the neighboring two RBDs, rendering it even less accessible by 40%.
Other epitopes repeat this pattern, but some make up part of the epitope itself, which increases the area unshielded, and leaves it exposed even in the ‘down’ conformation. Others are shielded heavily in both positions.
Glycosylation and Structural Stability of RBD in Up Position
The epitopes also play a major role in the structural stabilization of the RBD in the ‘open,’ ‘closed’ and mutant configurations, allowing the protein to remain ‘up’ and severely destabilizing it if even a single mutation is present. The movement of the protein is also affected by the presence of these N-glycans, being either a spinning motion centered on the spike helices or titling away or towards them. These movements will affect the opening of the RBD and expose hidden epitopes.
Analysis of the hydrogen bonding patterns in these configurations shows that many of the N-glycans interact deeply into various pockets, including that created by the opening of the RBD.
The study thus shows how the glycan shields a significant part of the surface of the S protein, changing with the conformation, and presenting varying degrees of exposure to antibodies and small molecules. The N-glycans at certain specific sites have other roles as well. Some of them modulate the conformational switching of the S protein and deciding the type of movement of the RBD. Overall, the researchers say, “Our work sheds new light on the full structure of this critical target and points to opportunities and challenges for small molecules and vaccine design.”
*Important Notice
bioRxiv publishes preliminary scientific reports that are not peer-reviewed and, therefore, should not be regarded as conclusive, guide clinical practice/health-related behavior, or treated as established information.
"load" - Google News
June 15, 2020 at 10:00AM
https://ift.tt/2N44qVn
Glycans "lock and load" the SARS-CoV-2 spike protein for infection - News-Medical.Net
"load" - Google News
https://ift.tt/2SURvcJ
https://ift.tt/3bWWEYd
Bagikan Berita Ini
0 Response to "Glycans "lock and load" the SARS-CoV-2 spike protein for infection - News-Medical.Net"
Post a Comment